Research
We are interested in the spatiotemporal behavior of living system at the single cell and tissue level. Our research is focused on understanding the interplay between the biological functions and the mechanical properties, which is crucial to elucidate how cells are mechanically self-organized and assembled to form a tissue. The motivation for our study is to elucidate (1) cell-to-cell variability in cell mechanics, (2) spatiotemporal fluctuations of intercellular cytoplasm and cell membrane, (3) the role of mechanical stimuli on cell states, and (4) cell-cell mechanical interactions. Our main techniques for single cell and tissue measurements are scanning probe microscopy (SPM) including atomic force microscopy (AFM) and scanning ion conductance microscopy (SICM), which enables to probe the mechanical and dynamical properties of cells at nanoscale, micro-patterned substrates to regulate cell shape and size, and various optical techniques including confocal microscopy and time lapse imaging. Taking advantage of these techniques, we explore the fundamental principle of living cell system underlying physical properties of cell and tissue.
Quantifying tissue stiffness at single cell level
Tissue morphogenesis exhibits large changes in shape and size from micrometer to millimeter scales. the tissue stiffness at nanoscale has been measured by atomic force microscopy (AFM), but the precision of the AFM analysis for tissue sampels is unclear. We proposed a simple analytical equation regarding contact elastic theory with the consideration of the tissue surface gradient and demonstrated the determination of cell stiffness in developing embryo at single-cell resolution (Fujii et al. AIP Adv., 2019).
Tissue cells regulate the stiffness through the surrounding cell stiffness
The detailed understanding of traction force (tension) and stiffness at the single level is crucial to elucidate how the mechanical properties regulate cell biological functions(Roca-Cusachs et al. Nat. Cell Biol. 2017). For cell population (multicellular ) system, recently, the intercellular tension has been investigated in cell monolayer (Park et al. Nat. Mater., 2015), but the intracellular stiffness is still elusive. To address this question, using an extremely wide-range scan atomic force microscopy (AFM), we successfully measure the spatial distribution of cell stiffness in cell monolayer and found that the cell stiffness exhibited a long-range spatial correlation, indicating that cells regulate their stiffness by feeling the stiffness of neighboring cells (Fujii et al. Biophys. J., 2019).
High-speed mapping of cell rheology: MULTI-FREQUENCY FORCE MODULATION AFM
The force-modulation atomic force microscopy (AFM) (Radmacher et al. Science, 1992) has been extensively used for measuring viscoelastic properties of living cells at a single frequency Living cells exhibit a frequency-dependent cell modulus, which follows a characteristic power-law behavior in the range of lower frequencies (<1 kHz) (Fabry et al. Phys. Rev. Lett., 2001), it is crucial to understand the detailed spatial distribution of the frequency-dependent cell modulus with AFM. The single-frequency force modulation AFM, however, has been difficult to map the frequency-dependent cell rheology due to the extremely long measurement time. Recently, we developed a multi-frequency force modulation AFM for mapping the frequency-dependent storage and loss moduli of living cells. The AFM technique will be useful for the visualization of intracellular mechanical structures and/or the diagnosis of cell diseases such as cancer (Takahashi and Okajima. Appl. Phys. Lett., 2015).

Cell-to-cell variation in cell mechanics: SINGLE CELL DIAGNOSTICS
The living cell is a compliant, viscoelastic material with a highly dynamic and continuously remodeling cytoskeleton. The rheological properties of adherent cells are mainly attributed to the cytoskeleton and are related to various cell functions. It is known among individual cells of the same source and type, the cell modulus exhibits a large log-normal distribution that is the result of spatial, temporal, and intrinsic variations. However, little is known about the origin of such large distributions, which complicate the statistical evaluation of pharmacological treatments and the comparison of different cell states. Our recent study revealed how this variation depends on the spatial location within the cell and on the actin filament cytoskeleton, the organization of which strongly influences cell mechanics. (P. Cai et al. Biophys. J, 2013)

Nanoscale fluctuation of cell membrane: ION CONDUCTANCE MICROSCOPY
Membranes are essential components of cells. Cell membranes are flexible and undergo dynamic morphological changes according to biological functions, and thus the characterization of cell surface fluctuations is crucial for a better understanding of cell function in relation to cell dynamics. Recently we succeeded to measure nanoscale fluctuations on the apical surfaces of epithelial cells connected to neighboring cells by scanning ion conductance microscopy (SICM). We found that the apparent fluctuation amplitude of cell membrane increased towards the cell center, suggesting that apical surface fluctuations are highly constrained at the cell–cell interface, in the vertical direction to the surface and by the underlying actin filaments. (Y. Mizutani et al. Appl. Phys. Lett., 2013)
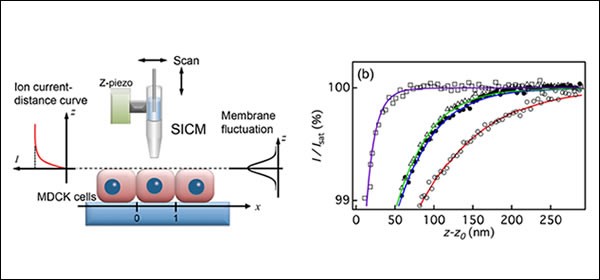
How do forces propagate through cell cytoskeleton? ATOMIC FORCE MICROSCOPY
When adherent cells contact a substrate, they form focal contacts, which are the adhesion sites at the cell–substrate interface via integrin receptors. The cells then form stress fibers that are made of a coalescence of actin filaments to anchor at the focal contacts and increase stiffness in response to stress applied to the integrins. To understand how forces between focal adhesions are propagated through actin filaments, the direct measurement of force propagation between focal adhesions of fibroblast cells was performed by mechanically modulating single cells by an atomic force microscopy (AFM) probe bound to cell apical surfaces where the microposts served as a force sensor at basal surfaces. The observation indicated that the force propagation from the apical to the basal surface exhibited no apparent out-of-phase response, indicating that the dynamic force propagating through cytoskeletal filaments behaves in an elastic manner. (A. Okada et al. Appl. Phys. Lett. (2011))
